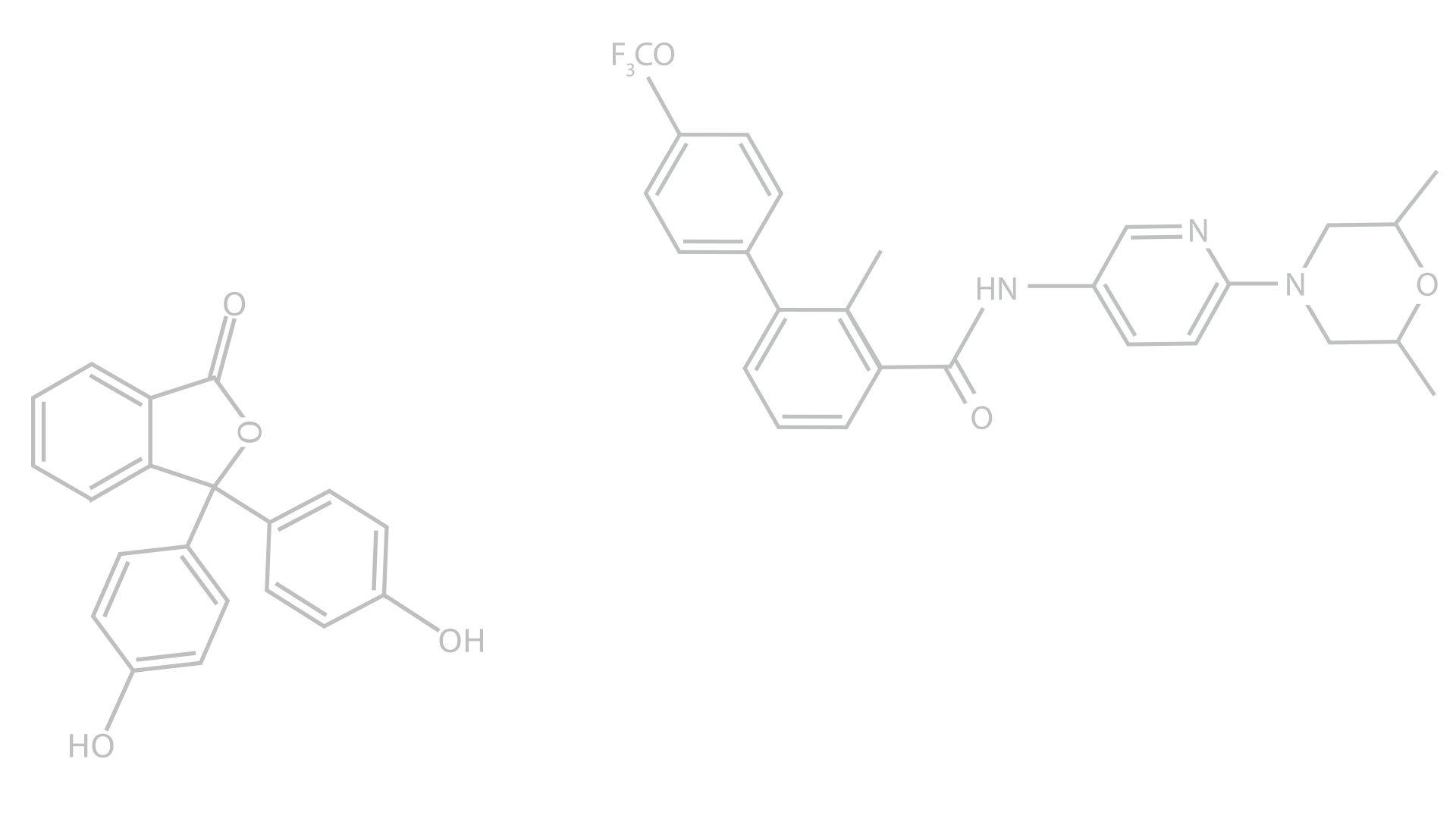

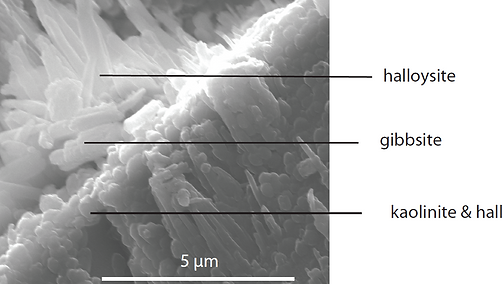
Clay Analysis via X-Ray Diffraction (XRD): A Comprehensive Guide
Introduction
Clay minerals, fine-grained hydrous phyllosilicates, are integral components of the Earth's crust and play crucial roles across various industries. These minerals, formed through weathering and low-temperature hydrothermal processes, possess unique properties that make them invaluable in applications ranging from mining and construction to geotechnical engineering and environmental remediation. This article provides an in-depth exploration of clay minerals, their significance, types, and the sophisticated analytical technique of X-Ray Diffraction (XRD) used to characterize them.
Industrial Significance of Clay Minerals
The distinctive characteristics of clay minerals, including high surface area, cation exchange capacity, and water retention properties, render them essential in numerous industrial applications:
Mining: Clay minerals can significantly impact mineral processing efficiency, particularly in flotation, settling, and filtration processes.
Construction and Geotechnical Engineering: Understanding clay mineralogy is crucial for soil stability assessment, foundation design, and the development of construction materials such as cement and ceramics.
Agriculture: Clay minerals influence soil fertility and water retention capacity.
Pharmaceuticals: Certain clay minerals are used as excipients and in drug delivery systems.
Environmental Remediation: Clays are employed in contaminant adsorption and waste containment.
Classification of Clay Minerals
Clay minerals comprise a diverse family, each type exhibiting distinct structural and chemical properties. The primary groups include:
Kaolinite: Characterized by low cation exchange capacity and limited swelling properties. Widely used in paper and ceramic industries.
Smectite (including Montmorillonite): Exhibits high cation exchange capacity and significant swelling properties. Valuable in applications such as drilling fluids, catalysts, and environmental remediation.
Illite: Possesses moderate cation exchange capacity and limited swelling. Commonly found in shales and important in the petroleum industry.
Chlorite: A non-swelling clay mineral with a unique chemical composition including magnesium, iron, and aluminum.
Accurate identification and quantification of these clay mineral types are essential for predicting their behavior and determining their suitability for specific applications.
X-Ray Diffraction (XRD) Analysis of Clay Minerals
X-Ray Diffraction is a powerful analytical technique that provides detailed information about the crystallographic structure and composition of clay minerals. By measuring the intensity and angles of diffracted X-rays, XRD can identify and quantify the different clay mineral types present in a sample.
Sample Preparation
Proper sample preparation is critical for accurate and reliable XRD analysis of clay minerals. The process typically involves the following steps:
Disaggregation: The bulk sample is gently crushed and disaggregated to separate individual particles without altering their crystalline structure.
Removal of cementing agents: Carbonates, organic matter, and iron oxides are removed using appropriate chemical treatments (e.g., acetic acid for carbonates, hydrogen peroxide for organic matter, and sodium dithionite-citrate-bicarbonate for iron oxides).
Dispersion: The sample is dispersed in deionized water, often with the addition of a dispersing agent such as sodium hexametaphosphate to prevent flocculation.
Size fractionation: The clay fraction (typically <2 μm) is separated from the bulk sample using sedimentation techniques based on Stokes' Law. This can be achieved through: a. Gravity settling: The suspension is allowed to settle, and the clay fraction is extracted at a calculated time and depth. b. Centrifugation: For faster separation, the suspension is centrifuged at a specific speed and duration to isolate the clay fraction.
Millipore filtration: After settling or centrifugation, the clay fraction (<2 μm) is passed through a filter paper using the Millipore method. This step ensures the capture of the desired clay-sized particles and removes any remaining larger particles or impurities.
Oriented mount preparation: The clay fraction collected on the filter paper is then used to create an oriented mount, which enhances the basal reflections of clay minerals. This can be done by: a. Smear mount: A small amount of clay from the filter paper is carefully smeared directly onto a glass slide. b. Transfer method: The filter paper containing the clay fraction is inverted onto a glass slide, and gentle pressure is applied to transfer the clay onto the slide surface. c. Suction method: If the clay was not initially captured on a filter paper, it can be drawn through a porous ceramic tile, depositing a thin, oriented layer on the surface.
Treatments: As outlined by the United States Geological Survey (USGS), several treatments are applied to enhance the identification of specific clay minerals:
Air-drying: To remove excess moisture and reveal basal reflections.
Ethylene glycol solvation: Expands the interlayer spacing of smectite clays. Samples are exposed to ethylene glycol vapor for at least 8 hours at 60°C.
Heat treatments:
400°C for 1 hour: Differentiates kaolinite from chlorite.
550°C for 1 hour: Further collapses kaolinite structure and identifies heat-resistant clay minerals. d.
Formamide treatment: Differentiates halloysite from kaolinite by exposing samples to formamide.
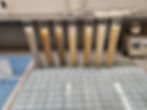
XRD Data Collection and Analysis
XRD patterns are collected using a diffractometer equipped with a copper X-ray tube. The diffraction patterns are then analyzed to identify clay minerals based on their characteristic reflections. Specialized software is often used to assist in peak identification and quantification.
The United States Geological Survey (USGS) has developed a comprehensive flow chart for clay mineral identification using XRD, which serves as a valuable reference for interpreting diffraction patterns. This chart outlines how different treatments affect the diffraction patterns of various clay minerals, allowing for their systematic identification.
Effects of treatments on XRD patterns:
Air-dried samples:
Provide a baseline diffraction pattern for comparison with treated samples.
Show the natural d-spacing of clay minerals under ambient conditions.
Ethylene glycol solvation:
Expands the interlayer spacing of smectite clays, shifting their (001) reflection from about 14 Å to 17 Å.
Helps distinguish smectites from other clay minerals that do not expand with glycolation.
Vermiculite may show a slight expansion, typically to about 14.5 Å.
Chlorite, kaolinite, and illite remain largely unaffected.
Heat treatment (400°C):
Collapses the structure of smectites, shifting their (001) reflection to about 10 Å.
Distinguishes kaolinite from chlorite: kaolinite's structure begins to break down, while chlorite remains stable.
Vermiculite collapses to about 10 Å, similar to smectites.
Illite remains largely unaffected.
Heat treatment (550°C):
Further collapses and may destroy the structure of kaolinite, causing its reflections to disappear.
Chlorite's (001) reflection at 14 Å may increase in intensity due to structural changes.
Smectites and vermiculites remain collapsed at about 10 Å.
Illite continues to be largely unaffected.
Formamide treatment:
Specifically used to distinguish halloysite from kaolinite.
Expands the halloysite structure to about 10.4 Å, while kaolinite remains unchanged at 7 Å.
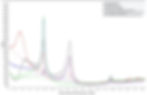
By comparing the diffraction patterns obtained from these various treatments, analysts can systematically identify the clay minerals present in a sample. For example:
A mineral that expands to 17 Å with glycolation and collapses to 10 Å upon heating is likely a smectite.
A mineral with a 7 Å peak that disappears upon heating to 550°C is likely kaolinite.
A mineral with a persistent 14 Å peak across all treatments is likely chlorite.
A mineral with a stable 10 Å peak across all treatments is likely illite.
The USGS flow chart provides a step-by-step guide for interpreting these patterns and identifying clay minerals based on their behavior under different treatments. This systematic approach, combined with knowledge of the sample's geological context and other analytical data, allows for accurate identification and characterization of clay minerals using XRD.
Quantification using Rietveld Analysis
The Rietveld method is a powerful approach for quantifying clay mineral abundances. This method involves fitting a calculated XRD pattern to the observed data by refining various structural and instrumental parameters. Specialized software, such as Siroquant, has been developed to facilitate Rietveld analysis of clay minerals, incorporating their unique structural and chemical properties into the refinement process.
Limitations and Complementary Techniques
While XRD is the gold standard for clay mineral analysis, it does have some limitations:
Difficulty in distinguishing between clay minerals with similar crystal structures (e.g., different smectite types).
Challenges in analyzing interstratified or mixed-layer clays.
Limited sensitivity for poorly crystalline or low-abundance clay minerals.
To overcome these limitations, complementary techniques may be employed:
Transmission Electron Microscopy (TEM): Provides high-resolution imaging and chemical analysis of individual clay particles.
Fourier Transform Infrared Spectroscopy (FTIR): Offers information on clay mineral structure and composition based on vibrational modes.
Thermal Analysis: Techniques such as Differential Thermal Analysis (DTA) and Thermogravimetric Analysis (TGA) can provide information on clay mineral dehydration and decomposition behavior.
Chemical Analysis: Techniques like X-ray Fluorescence (XRF) or Inductively Coupled Plasma Mass Spectrometry (ICP-MS) can provide elemental composition data to support XRD results.
Conclusion
X-Ray Diffraction remains an indispensable tool for clay mineral analysis, offering crucial insights into their structure, composition, and relative abundances. By employing rigorous sample preparation techniques, leveraging advanced analytical methods like Rietveld analysis, and complementing XRD with other analytical techniques, researchers and industry professionals can gain a comprehensive understanding of clay mineralogy. This knowledge is essential for optimizing the use of clay minerals across various applications and industries.